Hematopoietic stem cell transplantation (HSCT) refers to the process in which normal hematopoietic cells (HC) from a donor or the patient’s own body are infused into the patient following preconditioning with total-body irradiation, chemotherapy, and immunosuppression. This procedure aims to restore normal hematopoietic and immune functions. Hematopoietic cells (including hematopoietic stem cells [HSC] and progenitor cells) express the CD34 antigen. HSCs possess the ability to proliferate, differentiate, and self-renew, ensuring lifelong hematopoiesis.
Over the course of more than 60 years of development, HSCT has become a crucial clinical treatment option, with the number of transplant cases worldwide increasing annually. Patients who undergo transplantation have been reported to achieve disease-free survival for over 30 years. In 1990, Dr. E. Donnall Thomas was awarded the Nobel Prize in Physiology or Medicine for his groundbreaking contributions to bone marrow transplantation.
Classification
HSCT is classified based on whether the hematopoietic cells are obtained from a healthy donor or from the patient. These classifications include allogeneic HSCT and autologous HSCT (auto-HSCT). Allogeneic HSCT can be further subdivided into allogeneic hematopoietic stem cell transplantation (allo-HSCT) and syngeneic hematopoietic stem cell transplantation, with the latter referring to transplants between genetically identical monozygotic twins. In syngeneic transplantation, immunological issues such as graft rejection and graft-versus-host disease (GVHD) do not occur, but the probability of finding such matches is less than 1%.
HSCT can also be categorized based on the source of HSCs into:
- Bone marrow transplantation (BMT)
- Peripheral blood stem cell transplantation (PBSCT)
- Cord blood transplantation (CBT)
Another classification differentiates between related donor transplantation and unrelated donor transplantation (UDT), depending on whether the donor and recipient share a familial relationship.
In terms of human leukocyte antigen (HLA) matching, HSCT can be classified as HLA-matched, partially matched, or haploidentical transplantation.
Human Leukocyte Antigen Typing
The HLA gene complex, also known as the major histocompatibility complex (MHC), is located on the short arm of chromosome 6 (6p21) and exhibits extensive genetic and structural diversity. The HLA antigens most relevant to HSCT include the class I antigens (HLA-A, HLA-B, HLA-C) and the class II antigens (HLA-DR, HLA-DQ, HLA-DP). HLA mismatch significantly increases the risk of GVHD and host-versus-graft reaction (HVGR).
HLA haplotypes are inherited as a unit, meaning siblings have a 25% chance of being HLA-identical. Historically, HLA typing was performed using serological methods, but DNA-based genetic typing is now more commonly used.
For unrelated donor matches, high-resolution molecular techniques are essential. High-resolution HLA genotyping is expressed using at least four-digit numbers (e.g., A0101 vs. A0102). The first two digits represent the serologically detectable antigen (referred to as low-resolution), while the last two digits specify alleles that differ at the DNA sequence level (referred to as high-resolution). Previously, low-resolution typing was archived for unrelated donors, with high-resolution typing performed as needed. More recently, the proportion of high-resolution HLA data included at the time of donor registration in bone marrow registries has increased significantly.
Donor Selection
For auto-HSCT, the donor is the patient, who must be able to tolerate high-dose chemotherapy or radiotherapy and have sufficient hematopoietic stem cells that are not contaminated by tumor cells.
In general, for allo-HSCT, the preferred donor is an HLA-matched sibling. If such a donor is unavailable, HLA-matched unrelated donors (MUD), haploidentical related donors, or cord blood stem cells are considered as alternative options. Among multiple HLA-compatible donors, selection criteria include younger age, overall health, male, cytomegalovirus (CMV) seronegativity, and compatibility in red blood cell blood type.
Collection of Hematopoietic Cells
Donors for allo-HSCT need to be healthy individuals, with evaluations conducted to rule out infectious and chronic systemic diseases that would disqualify them from donating. Informed consent is required. The process of hematopoietic stem cell donation is safe, does not reduce the donor's immunity, and has no adverse impact on their health. Medical equipment and materials used during the collection process are single-use to prevent disease transmission.
Bone Marrow
The collection of bone marrow is a well-established routine procedure. It is commonly performed under continuous epidural anesthesia or general anesthesia, with aspiration typically done from both posterior superior iliac spines. The target collection is generally a nucleated cell count of (4–6)×108/kg, based on the recipient’s body weight. To maintain the donor's hemodynamic stability and ensure their safety, autologous blood is often collected 14 days before the procedure for reinfusion during the operation. When there is a blood type mismatch between the donor and recipient, red blood cells and/or plasma in the bone marrow must be removed to prevent an acute hemolytic reaction. For autologous BMT, collected bone marrow requires the addition of cryoprotectants for preservation. It is stored in liquid nitrogen or at -80°C in ultra-low temperature freezers and is thawed immediately prior to infusion during transplantation.
Peripheral Blood
Under normal conditions, the concentration of hematopoietic cells in peripheral blood is very low. Mobilization with granulocyte-colony stimulating factor (G-CSF) is required to increase CD34+ hematopoietic cells in the blood. The commonly used dosage is G-CSF at 5–10 µg/(kg·d), administered subcutaneously once or twice daily for four days. On the fifth day, a blood cell separator is used for collection. A minimum of 2×106 CD34+ cells per kilogram of recipient body weight is required to ensure rapid and stable hematopoietic recovery.
For recipients undergoing auto-PBSCT, chemotherapy with agents such as cyclophosphamide (CTX) or etoposide (VP-16) can be performed prior to collection to further eliminate tumor cells. Hematopoietic stem cells are then mobilized and collected during the recovery phase of leukocytes. The storage methods for autologous peripheral blood hematopoietic stem cells are similar to those used for bone marrow.
Cord Blood
The collection and storage of cord blood hematopoietic cells are managed by specialized cord blood banks. Before collection, the newborn must be confirmed to have no genetic diseases. Specimens are retained for blood typing, HLA matching, nucleated cell and CD34+ cell counts, and testing for various pathogens to ensure the quality of the cord blood.
Conditioning Regimens
The goals of conditioning regimens are:
- To eliminate the underlying disease as completely as possible.
- To suppress the recipient’s immune system to prevent graft rejection.
Conditioning involves the use of total-body irradiation (TBI), cytotoxic drugs, and immunosuppressive agents. Based on the intensity of the conditioning, HSCT can be classified into myeloablative HSCT (conventional HSCT) and non-myeloablative HSCT (NST). Reduced-intensity conditioning (RIC) HSCT falls between the two.
In NST, the conditioning regimen exerts reduced direct cytotoxicity on tumor cells. Instead, it primarily induces immune tolerance in the recipient, allowing donor cells to engraft and establish a stable chimerism. Immunologically active cells introduced with the graft or arising from the proliferation and differentiation of transplanted HSCs, as well as subsequent donor lymphocyte infusions (DLI), play an essential role in the graft-versus-leukemia (GVL) effect, ultimately contributing to the eradication of the tumor. NST is typically considered for patients with slow disease progression, relatively low tumor burden, and high sensitivity to the GVL effect, particularly those unsuitable for conventional transplantation or older adults (over 50 years of age). Fludarabine is commonly included in NST regimens.
For most patients, especially younger individuals with malignant diseases, conventional myeloablative conditioning remains the standard. Common conditioning regimens include:
- Fractionated TBI with a total dose of 12 Gy and CTX at 60 mg/(kg·d) for two consecutive days.
- Intravenous busulfan at 0.8 mg/(kg·6h) for four consecutive days combined with CTX at 60 mg/(kg·d) for two days.
- The BEAM regimen (BCNU + VP-16 + Ara-C + melphalan), used for lymphomas.
- The high-dose melphalan (HD-Mel) regimen (Mel 200 mg/m2), used for multiple myeloma (MM).
In autologous and syngeneic transplantations, where there is no GVL effect, the conditioning dose should be maximized, with agents selected to achieve synergistic pharmacological effects while minimizing overlapping toxicities.
Evidence of Engraftment and Component Transfusion
From the day of bone marrow transplantation (BMT), neutrophil counts typically recover to >0.5×109/L within four weeks, while platelet counts often take longer than four weeks to reach ≥50×109/L. The use of G-CSF at a dose of 5 μg/(kg·d) can shorten the duration of neutropenia by 5 to 8 days.
In peripheral blood stem cell transplantation (PBSCT), hematopoietic recovery is faster, with neutrophil and platelet recovery typically occurring 8–10 days and 10–12 days after transplantation, respectively. In contrast, cord blood transplantation (CBT) has slower hematopoietic recovery, with neutrophil recovery often taking more than one month, and platelet recovery taking even longer. Approximately 10% of CBT cases fail to achieve engraftment. On the other hand, HLA-matched BMT and PBSCT have engraftment rates as high as 97%–99%. The occurrence of graft-versus-host disease (GVHD) serves as clinical evidence of engraftment. Laboratory evidence can be obtained through cytological and molecular genetic methods (e.g., FISH technology) or through red and white blood cell antigen conversion experiments, based on differences in gender, blood type, or HLA between donor and recipient. For cases where donor and recipient are completely matched, techniques such as short tandem repeat (STR) and single nucleotide polymorphism (SNP) analysis combined with PCR can be used for confirmation.
Before hematopoietic recovery is achieved, HSCT recipients require transfusion support with blood components. Red blood cells are transfused when hematocrit drops to ≤0.30 or hemoglobin (Hb) levels decrease to ≤70 g/L. Platelets are transfused if there is bleeding with thrombocytopenia or when platelet counts are ≤20×109/L without bleeding (some institutions use a threshold of ≤10×109/L).
To prevent transfusion-associated GVHD, all blood products containing cellular components must be irradiated with 25–30 Gy to inactivate lymphocytes. The use of leukocyte filters can prevent febrile reactions, ineffective platelet transfusions, GVHD and host-versus-graft reaction (HVGR), transfusion-related acute lung injury, and can reduce the transmission of blood-borne CMV, EBV, and HTLV-I.
Complications
The complications of HSCT and their prevention and management are critical factors influencing the success of transplantation. The occurrence of complications is primarily related to the toxic side effects of high-dose radiochemotherapy, as well as immune suppression and dysregulation in post-transplant patients. Although the causes of most complications are well understood, for some complications, multiple factors contribute to their pathogenesis. Furthermore, patients may present with multiple concurrent complications. The incidence and severity of complications are significantly higher in allo-HSCT compared to auto-HSCT.
Toxicities from Conditioning Regimens
Different conditioning regimens result in varying toxic side effects. Early toxicities often include gastrointestinal reactions such as nausea, vomiting, and mucositis, as well as acute damage to liver and kidney function, and cardiovascular toxicity. Glucocorticoids can alleviate radiation-induced gastrointestinal injuries. Oral mucositis commonly occurs 5–7 days after transplantation and may require opioid analgesics in severe cases. Secondary herpesvirus infections are treated with acyclovir and intravenous nutritional support, with spontaneous resolution typically occurring within 7–12 days. Hair loss begins around 5–6 days after transplantation. Seizures caused by busulfan can be effectively prevented with clonazepam or phenytoin sodium. Hemorrhagic cystitis caused by high-dose CTX can be managed with mesna, adequate hydration, urinary alkalization, bladder irrigation, and transfusion support.
Long-term survivors of HSCT may experience late-onset complications related to conditioning, including:
- Cataracts: Primarily associated with TBI, with glucocorticoids increasing the risk.
- Leukoencephalopathy: Generally observed in patients with CNS leukemia who undergo repeated intrathecal chemotherapy combined with high-dose systemic radiochemotherapy.
- Endocrine Disorders: Conditions such as hypothyroidism, hypogonadism, amenorrhea, azoospermia, infertility, and delayed growth in children may occur.
- Secondary Malignancies: A small percentage of patients develop lymphoma or other solid tumors several years after transplantation; secondary leukemia or myelodysplastic syndromes (MDS) may also arise.
Infections
Infections are relatively common after HSCT due to pancytopenia, neutropenia, indwelling catheters, damage to mucosal barriers, and impaired immune function. Infection prevention measures typically include:
- Protective isolation, such as housing patients in laminar airflow clean rooms.
- A sterile diet.
- Gastrointestinal decontamination.
- Intravenous immunoglobulin support.
- Emphasis on personal hygiene for patients, family members, and healthcare workers, including frequent handwashing and wearing masks.
Infections after HSCT are generally divided into three phases:
- Early Phase: Within the first month after transplantation.
- Intermediate Phase: From one month to 100 days after transplantation.
- Late Phase: Beyond 100 days after transplantation.
Each phase is characterized by different infection risks and pathogenic microorganisms. Infection risk in the later stages depends on the degree of immune system recovery.
Bacterial Infections
The early phase presents the highest risk due to multiple susceptibility factors. Fever may be the only sign of infection, often without typical inflammatory symptoms and signs. Treatment should adhere to guidelines for managing infections in high-risk neutropenic patients, beginning with broad-spectrum, high-dose intravenous antibiotics as soon as possible. Blood cultures and pathogen detection from suspected infection sites should be conducted to guide adjustments in therapy based on infection type, pathogen identification, and local bacterial resistance patterns in the healthcare facility.
During the intermediate and late phases, while bone marrow hematopoietic function may have recovered, immune function remains compromised. Patients with GVHD or hypogammaglobulinemia are at particularly high risk of infection during this time.
Viral Infections
Herpesvirus infections are the most common viral infections after transplantation. Infections caused by herpes simplex virus respond well to intravenous treatment with acyclovir at 5 mg/kg every 8 hours. For prophylaxis, oral acyclovir at reduced doses can be utilized. To prevent late reactivation of varicella-zoster virus, which has an activation rate between 40% and 60%, acyclovir can be extended for up to one year after transplantation. Infections caused by Epstein-Barr virus (EBV) and human herpesvirus 6 (HHV-6) are also relatively common, with EBV being closely associated with post-transplant lymphoproliferative disorders and HHV-6 linked to encephalitis.
Cytomegalovirus (CMV) infection is the most severe viral complication after transplantation, typically occurring during the intermediate to late post-transplant phase. CMV infection may result from viral reactivation in the patient or transfusion of CMV-positive blood products. Patients who are CMV-negative (both donor and recipient) should only receive CMV-negative blood products. Clinical manifestations of CMV infection include interstitial pneumonia (IP), CMV colitis, CMV hepatitis, and CMV retinitis. Treatment for CMV infection typically involves supportive care and antiviral therapy, with agents such as ganciclovir or foscarnet being recommended.
Fungal Infections
Administration of fluconazole at 400 mg/day orally has significantly reduced the incidence of Candida albicans infections. However, the incidence of other fungal infections has risen noticeably in recent years. Treating invasive fungal infections, particularly aspergillosis and mucormycosis, remains a significant challenge. Depending on the diagnosis, antifungal drugs such as itraconazole, voriconazole, caspofungin, micafungin, or amphotericin B are commonly used.
Pneumocystis jirovecii Pneumonia
Prophylaxis for Pneumocystis jirovecii pneumonia typically involves the administration of trimethoprim-sulfamethoxazole (SMZco; 160 mg of trimethoprim and 800 mg of sulfamethoxazole) starting one week prior to transplantation. The regimen often involves taking the medication twice daily for two days each week, continuing until the cessation of immunosuppressive therapy. This approach has proven highly effective in prevention.
Hepatic Sinusoidal Obstruction Syndrome (HSOS)
Hepatic sinusoidal obstruction syndrome, formerly known as hepatic veno-occlusive disease, may result from endothelial damage caused by the transplantation process. Other clinical syndromes, such as engraftment syndrome, capillary leak syndrome, diffuse alveolar hemorrhage, and thrombotic microangiopathy, may also arise. The clinical features of HSOS include unexplained weight gain, jaundice, right upper quadrant pain, hepatomegaly, and ascites. The incidence is approximately 10%, and liver biopsy is required for definitive diagnosis. The primary causes include cytotoxic injury to hepatic vasculature and sinusoidal endothelial cells, as well as a localized hypercoagulable state. HSOS most commonly occurs within two weeks after transplantation, with most cases arising within one month.
Risk factors for HSOS are typically divided into patient-related and transplant-related categories. Patient-related risk factors include age, physical condition, pre-existing liver disease or abnormal liver function, disease progression, thalassemia, iron overload, prior abdominal radiotherapy, and the use of agents such as gemtuzumab or inotuzumab ozogamicin. Transplant-related factors include allo-HSCT (compared to auto-HSCT), HLA mismatch or haploidentical transplantation, second transplantation, grafts not processed for T-cell depletion, conditioning regimens that include busulfan (BU) or TBI, as well as GVHD prophylaxis involving fludarabine, calcineurin inhibitors, and sirolimus. Prophylaxis methods, including low-dose heparin [100 U/(kg·d) continuous intravenous infusion for 30 days], prostaglandin E1, and ursodeoxycholic acid, have demonstrated effectiveness in reducing the incidence of HSOS.
Treatment for mild to moderate HSOS primarily involves supportive care, including sodium restriction, microcirculation improvement, and diuretic therapy. Such cases often resolve spontaneously without sequelae. Severe cases, however, carry a poor prognosis, with death often resulting from progressive acute liver failure, hepatorenal syndrome, or multiorgan failure.
Graft-Versus-Host Disease (GVHD)
Graft-versus-host disease is a unique complication of allo-HSCT and a major cause of transplantation-related mortality. It arises from donor T cells attacking alloantigens of the recipient. Three key conditions are necessary for the occurrence of GVHD:
- The graft must contain immunologically active cells.
- The recipient must express tissue antigens not present in the donor.
- The recipient must be in an immunosuppressed state, unable to reject the graft.
Even with complete HLA matching between donor and recipient, discrepancies in minor histocompatibility antigens may still lead to GVHD, with severe GVHD occurring in approximately 30% of cases. Risk factors for GVHD include the degree of HLA matching, whether the donor and recipient are related, differences in sex, age, underlying disease and its status, conditioning regimens, GVHD prophylaxis protocols, graft characteristics, infections, and tissue damage.

Table 1 Classification of graft-versus-host disease (GVHD)
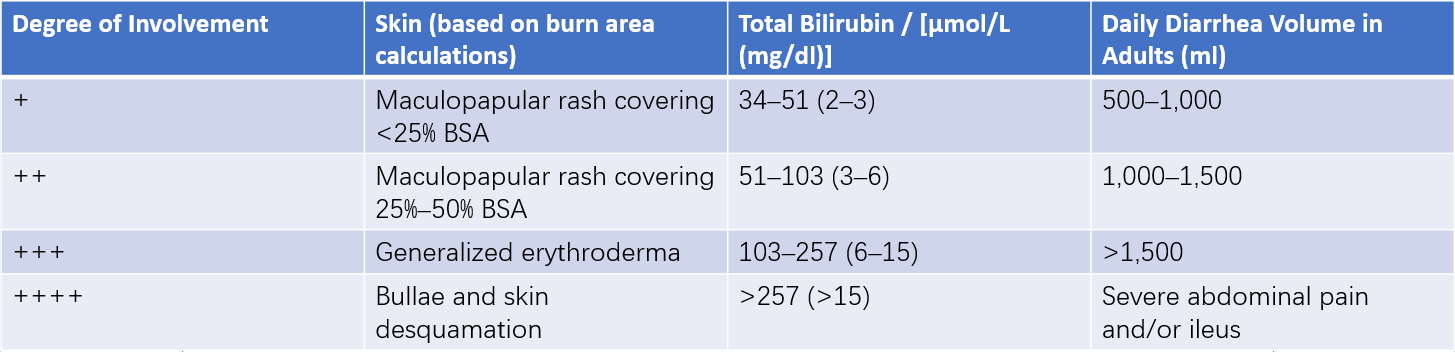
Table 2 Degree of organ involvement in acute GVHD

Table 3 Clinical grading of acute GVHD
GVHD can be classified into acute GVHD (aGVHD) and chronic GVHD (cGVHD). Typical aGVHD occurs within 100 days after transplantation, while cGVHD develops after 100 days. However, current practice emphasizes clinical presentation over timing for diagnosis. aGVHD primarily affects the skin, gastrointestinal tract, and liver. Common manifestations include skin erythema and maculopapular rashes, persistent anorexia and/or diarrhea, and abnormal liver function. While tissue biopsies aid in diagnosis, clinical judgment remains paramount to avoid delays in treatment.
The clinical severity of aGVHD is graded from I to IV. Grade I generally does not require systemic therapy, while grades II to IV significantly affect survival and prognosis, necessitating rapid and aggressive intervention. Treatment outcomes for aGVHD are often suboptimal, making prevention particularly important. Common preventive strategies include the use of immunosuppressive agents and T-cell depletion. A standard prophylactic regimen typically involves cyclosporine (CsA) combined with methotrexate (MTX).
CsA inhibits calcineurin, thereby blocking the transcription of the IL-2 gene and subsequently preventing IL-2-dependent T-cell proliferation and differentiation. Initial administration involves intravenous infusion at 2–4 mg/(kg·d), transitioning to oral administration once gastrointestinal side effects subside, with the blood concentration maintained at 150–250 ng/ml. CsA therapy is discontinued if serum creatinine exceeds 177 μmol/L (2.0 mg/dl). The dosage is typically reduced weekly by 5% starting 40 days after transplantation and is generally administered for at least six months. Methotrexate (MTX) is delivered via intravenous infusion on day 1 (15 mg/m2), followed by doses on days 3, 6, and 11 (10 mg/m2 each). Additional options for prophylaxis include tacrolimus (FK506) and mycophenolate mofetil (MMF).
For haploidentical transplantation, approaches such as the use of G-CSF and ATG in non-ex vivo T-cell depletion transplantation or post-transplant cyclophosphamide have effectively reduced the incidence of acute GVHD (aGVHD). Direct T-cell removal from the graft also prevents GVHD but may increase the risks of graft failure, relapse, and infections after transplant.
Severe aGVHD is challenging to treat. The primary treatment is methylprednisolone at 1–2 mg/(kg·d). Second-line options include anti-IL-2 receptor (IL-2RA) monoclonal antibodies, MTX, ruxolitinib, MMF, tacrolimus, sirolimus, ATG, and other agents. Novel therapies, such as fecal microbiota transplantation, have also been applied.
Chronic GVHD (cGVHD) develops in approximately 30%–70% of patients who survive more than six months after transplantation. Risk factors for cGVHD include older age, HLA mismatch, unrelated donor transplantation, PBSCT, and a history of aGVHD. cGVHD can affect all organs and tissues, presenting clinical features similar to autoimmune diseases. The primary treatment involves glucocorticoids, with or without calcineurin inhibitors, with concurrent infection prevention strategies.
Post-Transplant Relapse
Relapse occurs in some patients after transplantation, with the probability influenced by factors such as disease risk stratification, disease status at transplantation, and transplant type. Most relapses occur within three years after transplantation, and treatment outcomes are typically unsatisfactory, with a poor prognosis. Monitoring for minimal residual disease helps identify high-risk patients with persistently elevated or increasing levels. For these patients, ways to potentially reduce relapse rates include adjusting the intensity of immunosuppressive therapy, combining donor lymphocyte infusion (DLI), infusing CAR-T cells, or prophylactic application of molecular targeted therapies. In some cases, a second transplantation may be suitable for relapsed patients.
Primary Indications
As HSCT techniques and treatments for associated diseases continue to advance, indications for HSCT are continuously updated. The upper age limit for patients has gradually been extended, and non-myeloablative stem cell transplantation (NST) is not typically restricted by age. The timing and type of transplant for each patient are guided by treatment protocols and individual clinical conditions.
Non-Malignant Diseases:
- Severe aplastic anemia (SAA): HSCT is the preferred option for patients younger than 50 years of age with severe or very severe aplastic anemia who have HLA-matched sibling donors.
- Paroxysmal nocturnal hemoglobinuria (PNH): Particularly in patients with accompanying features of aplastic anemia.
- Other diseases: HSCT can treat congenital hematopoietic disorders and metabolic diseases caused by enzyme deficiencies, such as Fanconi anemia, sickle cell anemia, severe thalassemia, and severe combined immunodeficiency. Research is ongoing for its role in treating refractory acquired autoimmune diseases.
Malignant Diseases:
- Malignant hematological diseases: HSCT, especially allo-HSCT, is an effective treatment for hematologic malignancies (details can be found in chapters on specific diseases). Generally, acute myeloid leukemia (AML), acute lymphoblastic leukemia (ALL), and myelodysplastic syndromes (MDS) are treated with allogeneic transplantation. Lymphomas and multiple myelomas are often treated with autologous transplantation, though allogeneic transplantation is also an option.
- Solid tumors sensitive to radiotherapy and chemotherapy: Autologous HSCT may be considered for certain solid tumors.
Quality of Life and Outlook
The successful application of HSCT has enabled long-term survival for many patients. Most survivors experience good physical and psychological health, allowing them to return to normal work, study, and daily life. Around 10%–15% of survivors encounter psychosocial challenges, with cGVHD being the primary factor affecting quality of life. Continued improvements in transplantation techniques, along with progress in related fields, are anticipated to allow HSCT to cure even more patients in the future.