Heart failure (HF) is a complex clinical syndrome resulting from structural and/or functional abnormalities of the heart, leading to impaired contraction and/or filling. This causes decreased cardiac output or increased intracardiac pressures at rest or during exercise. It is primarily manifested by reduced exercise tolerance and fluid retention. Cardiac dysfunction is a broader concept, and when it presents with clinical symptoms, it is termed heart failure.
Classification
Classification by occurrence site
Left heart failure is due to left ventricular dysfunction and is more common clinically. Right heart failure results from right ventricular dysfunction. When both left and right heart failure are present, it is termed global heart failure.
Classification by onset speed
Heart failure can be classified into chronic heart failure and acute heart failure based on the speed of onset.
Acute heart failure occurs due to acute severe myocardial damage, structural destruction, arrhythmias, or sudden increased cardiac load, leading to rapid deterioration of cardiac function in a previously normal or compensated heart. It can be an initial episode or an acute exacerbation of chronic heart failure.
Chronic heart failure has a slow progression, typically involving compensatory cardiac enlargement or hypertrophy and other compensatory mechanisms.
Classification by left ventricular ejection fraction (LVEF)
Classification by LVEF is clinically significant, especially for treatment guidance. LVEF ≤ 40% is classified as heart failure with reduced ejection fraction (HFrEF), traditionally known as systolic heart failure. LVEF of 41% - 49% is classified as heart failure with mildly reduced ejection fraction (HFmrEF), primarily involving mild systolic dysfunction. LVEF ≥ 50% is classified as heart failure with preserved ejection fraction (HFpEF), usually with elevated filling pressures and diastolic dysfunction, previously called diastolic heart failure. Most HFrEF patients also have diastolic dysfunction, and HFpEF patients may have systolic dysfunction.
It is important to note that LVEF is dynamic and should be regularly monitored throughout management. Previous LVEF ≤ 40% but LVEF > 40% during treatment are classified as heart failure with improved ejection fraction (HFimpEF).
Etiology
Primary etiology
Decreased myocardial contractility
Primary myocardial damage includes ischemic myocardial damage due to coronary artery disease such as myocardial infarction and chronic myocardial ischemia; inflammatory myocardial damage such as myocarditis; and genetic cardiomyopathies such as dilated cardiomyopathy, hypertrophic cardiomyopathy, and arrhythmogenic right ventricular cardiomyopathy.
Secondary myocardial damage is caused by endocrine and metabolic diseases (diabetes, thyroid disorders), systemic infiltrative diseases (cardiac amyloidosis), storage diseases (glycogen storage disease, α-galactosidase A deficiency), connective tissue diseases, and cardiotoxic drugs.
Restricted ventricular diastole and filling
Ventricular filling restriction occurs when cardiac diastole and filling are impaired due to heart disease, despite adequate venous return. For example, in hypertrophic cardiomyopathy, reduced compliance of the hypertrophied myocardium leads to impaired diastolic filling. In atrial fibrillation with a rapid ventricular rate, shortened diastole and atrioventricular asynchrony restrict ventricular filling, reducing stroke volume and causing systemic and pulmonary congestion.
Excessive cardiac load
Pressure overload (afterload) can be seen in conditions such as hypertension, aortic stenosis, pulmonary hypertension, and pulmonary valve stenosis, where left or right ventricular ejection resistance increases. Initially, compensatory myocardial hypertrophy occurs to handle the increased workload and maintain cardiac output. However, chronic excessive pressure overload can lead to heart failure if it exceeds myocardial compensatory capacity.
Volume overload (preload) is common in valvular insufficiency and congenital heart diseases with left-to-right or arteriovenous shunts, as well as conditions with increased blood volume or metabolic rate, such as chronic anemia, hyperthyroidism, and arteriovenous fistulas. Initially, compensatory ventricular dilation maintains cardiac output. However, prolonged volume overload can lead to heart failure if it surpasses myocardial compensatory capacity.
Precipitating factors
Infection
Infection is the most common precipitating factor. Fever from infection can increase heart rate and myocardial oxygen consumption. Respiratory infections, especially those with bronchospasm, mucosal congestion, and edema, can increase pulmonary circulation resistance, exacerbating right ventricular afterload.
Arrhythmias
Atrial fibrillation is one of the most common arrhythmias in organic heart disease and a significant precipitating factor for heart failure. Other types of tachyarrhythmias and severe bradyarrhythmias can also induce heart failure.
Increased cardiac preload and afterload
Excessive sodium intake and rapid or excessive intravenous fluid administration can increase cardiac preload, while elevated blood pressure increases afterload. Additionally, overexertion, pregnancy and childbirth, emotional stress, trauma, and surgery can increase cardiac load and trigger heart failure.
Improper treatment
During stable phases of heart failure, inappropriate discontinuation or reduction of heart failure medications can exacerbate ventricular remodeling, leading to deterioration of heart failure.
Exacerbation of existing heart conditions or comorbidities
This includes myocardial infarction in coronary artery disease, rheumatic activity in rheumatic heart disease, progression of cardiomyopathy, and complications such as renal insufficiency and anemia.
Pathophysiology
Heart failure begins with the impact of risk factors on the heart. Initially, normal cardiac output is maintained through the heart's own compensatory mechanisms and neurohormonal compensation. However, these compensatory mechanisms lead to further myocardial damage, exacerbating ventricular remodeling and ultimately resulting in decompensation and heart failure.
Intrinsic cardiac mechanisms
Frank-Starling mechanism
A normal ventricle can adjust its stroke volume over a wide range to meet the demands of exercise and stress. When cardiac preload increases, the volume of blood returning to the heart rises, leading to an increase in end-diastolic volume, which enhances myocardial contractility. This increases cardiac output and work.
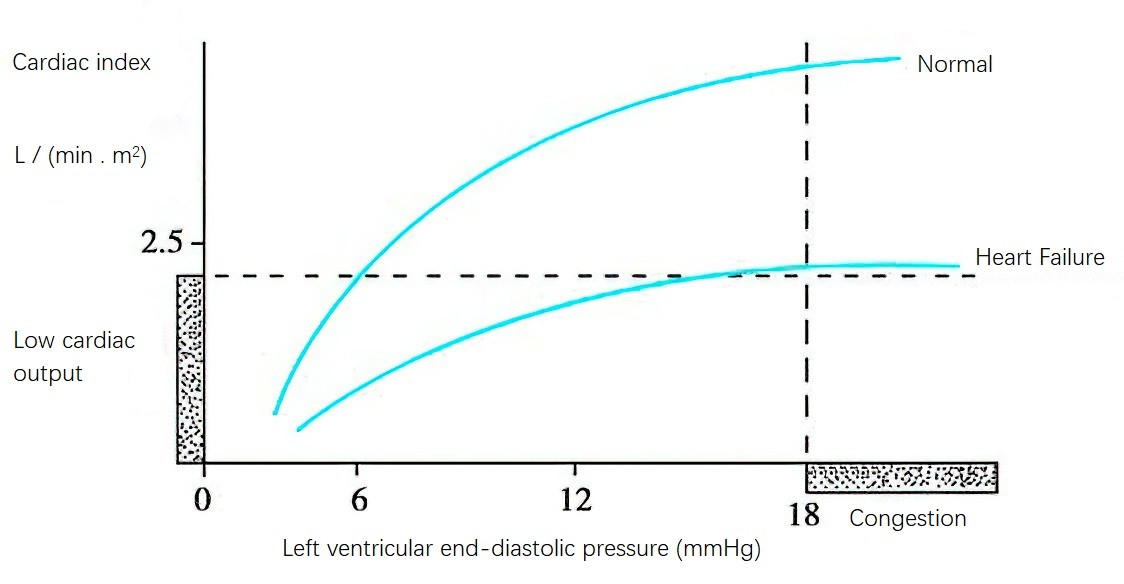
Figure 1 Left ventricular function curve
Systolic dysfunction
When myocardial contractility decreases, the enhanced ventricular contraction regulated by the Frank-Starling mechanism cannot maintain normal cardiac output. This leads to increased ventricular end-diastolic pressure and elevated atrial and venous pressures, and can eventually cause congestion in the pulmonary and/or systemic circulation.
Diastolic dysfunction
The importance of diastolic dysfunction in heart failure is increasingly recognized. It can generally be divided into two categories:
- Impaired energy-dependent processes of calcium reuptake into the sarcoplasmic reticulum and extrusion from the cell, leading to active diastolic dysfunction, commonly seen in myocardial ischemia, chronic volume overload, and aging
- Reduced ventricular compliance and filling impairment, mainly observed in ventricular hypertrophy and endocardial fibrosis, such as in hypertension and hypertrophic cardiomyopathy.
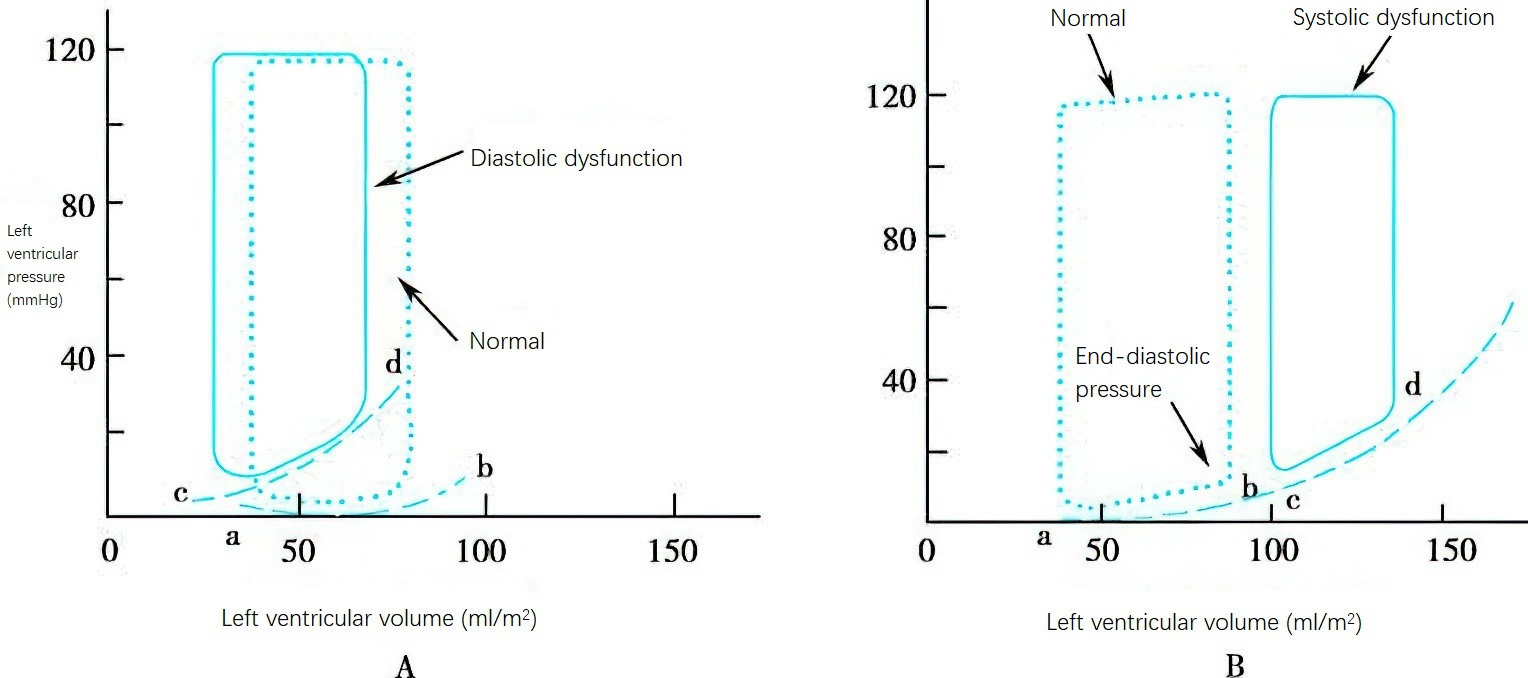
Figure 2 Changes in chamber pressure and volume with diastolic and contractile dysfunctions
A. Pure diastolic dysfunction: The pressure-volume curve shifts to the left, with a slight decrease in end-diastolic volume and a predominant increase in diastolic pressure; a - b, left ventricular diastolic pressure-volume change curve when cardiac function is normal; c - d, left ventricular diastolic pressure-volume change curve when diastolic function is impaired. B. Impaired contractile function: The pressure-volume curve shifts to the right, with significant increases in both systolic and diastolic volumes and an increase in end-diastolic pressure; a - b, left ventricular diastolic pressure-volume change curve when cardiac function is normal; c - d, left ventricular diastolic pressure-volume change curve when contractile function is impaired.
Neurohormonal mechanisms
When cardiac output is insufficient and intracardiac pressure rises, the body activates comprehensive neurohormonal compensatory mechanisms.
Sympathetic-adrenal medullary system enhancement
In heart failure patients, norepinephrine (NE) levels increase, acting on myocardial β1-adrenergic receptors to enhance myocardial contractility and increase heart rate, thereby improving cardiac output. NE also acts on α-receptors, causing vasoconstriction in peripheral and visceral resistance vessels, raising arterial blood pressure to ensure perfusion to the heart and brain. However, peripheral vasoconstriction increases cardiac afterload, and increased heart rate elevates myocardial oxygen consumption. NE also has direct toxic effects on myocardial cells, promoting apoptosis. Additionally, sympathetic nervous system excitation can enhance myocardial excitability, promoting arrhythmias.
Renin-angiotensin-aldosterone system (RAAS) enhancement
Reduced cardiac output decreases renal blood flow, excessively activating the RAAS. Angiotensin II (AT II) has a potent vasoconstrictive effect, working with NE to raise arterial blood pressure. Aldosterone increases circulating blood volume through sodium and water retention to improve cardiac output and ensure blood supply to vital organs like the heart and brain. However, excessive RAAS activation promotes hypertrophy or proliferation of myocardial and non-myocardial cells, and acts on cardiac fibroblasts to enhance collagen synthesis and cardiac fibrosis, thereby exacerbating ventricular and vascular remodeling and accelerating myocardial damage and deterioration of cardiac function.
Natriuretic peptide system enhancement
In heart failure, increased ventricular wall tension significantly raises brain natriuretic peptide secretion, which inhibits renal tubular sodium reabsorption and suppresses aldosterone and antidiuretic hormone secretion, promoting natriuresis and reducing cardiac volume load. Additionally, it counteracts the vasoconstrictive effects of AT II and inhibits renin secretion from juxtaglomerular cells.
Changes in other humoral factors
Besides the major neuroendocrine compensatory mechanisms, many other humoral regulatory factors are involved in heart failure. Arginine vasopressin (AVP) is released from the pituitary, promoting antidiuresis and peripheral vasoconstriction. In heart failure, the sensitivity of atrial stretch receptors decreases, failing to inhibit AVP release, leading to elevated plasma AVP levels. AVP causes systemic vasoconstriction through V1 receptors and reduces free water clearance through V2 receptors, increasing water retention and cardiac preload and afterload. In early heart failure, AVP has a compensatory effect, but long-term elevation exacerbates heart failure. Additionally, endothelin, nitric oxide, bradykinin, and various cytokines and inflammatory mediators are involved in the pathophysiology of chronic heart failure.
Ventricular remodeling
During the compensation process of impaired cardiac function, chamber enlargement, and myocardial hypertrophy, changes occur in myocardial cells, non-myocardial cells, extracellular matrix, and collagen fiber network, and are termed ventricular remodeling. This is a fundamental pathological mechanism in the development of heart failure. In addition to limited compensatory capacity and negative effects of compensatory mechanisms, inadequate energy supply and utilization in myocardial cells lead to necrosis and fibrosis, contributing significantly to decompensation. The reduction in myocardial cells decreases overall contractility, while increased fibrosis reduces ventricular compliance, exacerbating remodeling and forming a vicious cycle, ultimately leading to an irreversible terminal stage.
To be continued